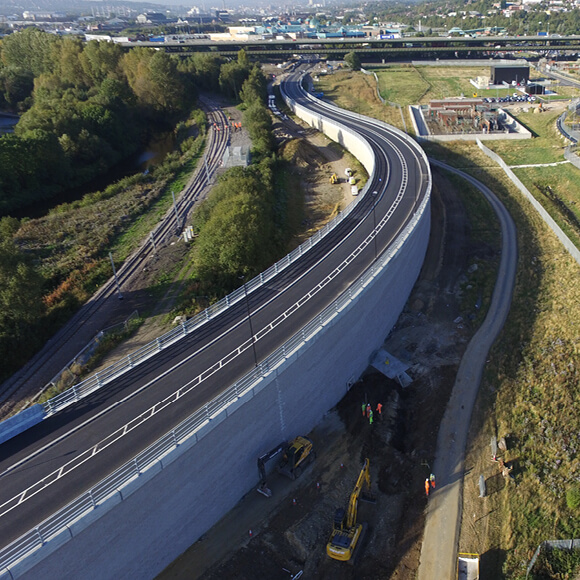
Retaining Wall Systems & Slope Stabilisation
Solution Overview
Wherever vertical separation is needed between adjacent finished ground levels on a project, the higher level must be supported either by an soil retaining wall system or by slope stabilisation. Naturally stable slopes take up a large footprint which is unavailable for development and may not be feasible due to space constraints. Retaining wall systems – either vertical retaining walls or steep reinforced slopes – can be constructed with a greatly reduced footprint to support the higher level platform.
Tensar offers a range of TensarTech Earth Retaining Systems for soil retaining walls and steep reinforced soil slopes. They have many advantages over alternative retaining wall systems such as reinforced concrete or masonry walls. They are faster to construct and considerably cheaper to build with a lower carbon cost.
Aesthetically Pleasing Solutions
TensarTech soil retaining wall systems have a choice of faces to suit local design codes, budget, environmental constraints and aesthetic requirements. TensarTech slope stabilisation systems can have a ‘green’ naturally vegetated face to enhance the local environment, while TensarTech retaining wall systems have face options that include moulded panels, smooth or rough textured blocks or natural rock fill.
Financially Attractive Solutions
The modular construction of TensarTech retaining wall solutions requires no large cranage or specialist on-site skills. In many cases the structural fill can be site-won material, suitable waste such as mine spoil or recycled aggregates. Each earth retaining wall system allows rapid and economical construction. Staged construction is easily accommodated, enabling flexible programming and reducing overall construction times.
Tensar's Expert Support
Tensar is the industry leader inground retaining systems and soiul retaining wall solutions. We've developed products and technologies at the forefront of the geotechnical industry for more than three decades. Our retaining wall products are backed by the most thorough quality assurance practices in the industry. And we can provide full engineering and construction support, including detailing, design, site assistance, and review of stamped drawings for each of our grade separation solutions.
How does Tensar compare to the competition?
Tensar's soil retaining wall and slope stabilisations offer flexibility, resilience, and cost savings that alternative mechanically stabilised earth (MSE) retaining wall systems and reinforced concrete retaining walls can't provide.